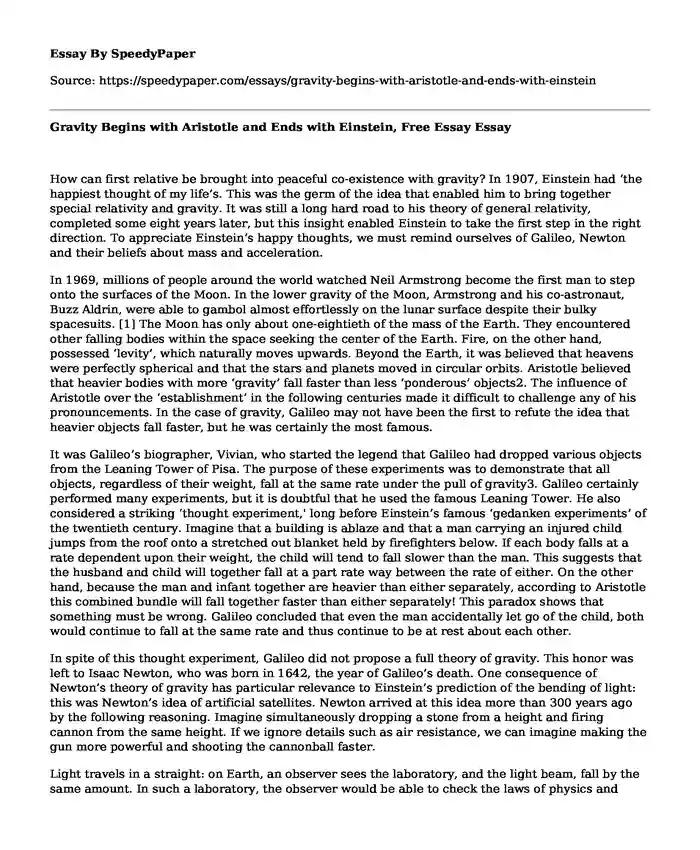
Type of paper: | Essay |
Categories: | History Physics Science |
Pages: | 7 |
Wordcount: | 1854 words |
How can first relative be brought into peaceful co-existence with gravity? In 1907, Einstein had ‘the happiest thought of my life’. This was the germ of the idea that enabled him to bring together special relativity and gravity. It was still a long hard road to his theory of general relativity, completed some eight years later, but this insight enabled Einstein to take the first step in the right direction. To appreciate Einstein’s happy thoughts, we must remind ourselves of Galileo, Newton and their beliefs about mass and acceleration.
In 1969, millions of people around the world watched Neil Armstrong become the first man to step onto the surfaces of the Moon. In the lower gravity of the Moon, Armstrong and his co-astronaut, Buzz Aldrin, were able to gambol almost effortlessly on the lunar surface despite their bulky spacesuits.[1] The Moon has only about one-eightieth of the mass of the Earth. They encountered other falling bodies within the space seeking the center of the Earth. Fire, on the other hand, possessed ‘levity’, which naturally moves upwards. Beyond the Earth, it was believed that heavens were perfectly spherical and that the stars and planets moved in circular orbits. Aristotle believed that heavier bodies with more ‘gravity’ fall faster than less ‘ponderous’ objects2. The influence of Aristotle over the ‘establishment’ in the following centuries made it difficult to challenge any of his pronouncements. In the case of gravity, Galileo may not have been the first to refute the idea that heavier objects fall faster, but he was certainly the most famous.
It was Galileo’s biographer, Vivian, who started the legend that Galileo had dropped various objects from the Leaning Tower of Pisa. The purpose of these experiments was to demonstrate that all objects, regardless of their weight, fall at the same rate under the pull of gravity3. Galileo certainly performed many experiments, but it is doubtful that he used the famous Leaning Tower. He also considered a striking ‘thought experiment,' long before Einstein’s famous ‘gedanken experiments’ of the twentieth century. Imagine that a building is ablaze and that a man carrying an injured child jumps from the roof onto a stretched out blanket held by firefighters below. If each body falls at a rate dependent upon their weight, the child will tend to fall slower than the man. This suggests that the husband and child will together fall at a part rate way between the rate of either. On the other hand, because the man and infant together are heavier than either separately, according to Aristotle this combined bundle will fall together faster than either separately! This paradox shows that something must be wrong. Galileo concluded that even the man accidentally let go of the child, both would continue to fall at the same rate and thus continue to be at rest about each other.
In spite of this thought experiment, Galileo did not propose a full theory of gravity. This honor was left to Isaac Newton, who was born in 1642, the year of Galileo’s death. One consequence of Newton’s theory of gravity has particular relevance to Einstein’s prediction of the bending of light: this was Newton’s idea of artificial satellites. Newton arrived at this idea more than 300 years ago by the following reasoning. Imagine simultaneously dropping a stone from a height and firing cannon from the same height. If we ignore details such as air resistance, we can imagine making the gun more powerful and shooting the cannonball faster.
Light travels in a straight: on Earth, an observer sees the laboratory, and the light beam, fall by the same amount. In such a laboratory, the observer would be able to check the laws of physics and special relativity is a situation with no gravity. Just as gravity can be ‘abolished’ by free-fall, an artificial gravity can be created by acceleration. As a rocket lifts off from the Launchpad, an astronaut feels heavier, and is pressed into the bunk by the so-called ‘g-forces.' Similarly, if an airplane suddenly begins to descend, you feel pulled out your seat. In the first case, some of the effects of gravity have been enhanced by acceleration: in the second case, some has been canceled. Gravity and acceleration are, in a certain sense, equivalent: this is the origin of Einstein’s new interpretation of Newton’s ‘equivalence principle’. To Newton, the ‘equivalence principle’ was the striking fact that the mass of a body that determined its response to a force the ‘inertia’ that governs its rate of acceleration appeared to be directly proportional to the weight of the body, which determines how strongly it reacts to a gravitational field, regardless of their mass. Newton was not content to accept such a coincidence as fact without performing some experimental tests. He constructed a pair of identical pendulums consisting of a wooden box at the end of an 11-foot wire. One box was then filled with wood, and the other was filled with the same weight of gold. If Newton’s ‘equivalence’ of ‘inertia’ mass and gravitational weight was correct, the period of the swings of the pendulums should depend only on the length of the wire suspending the boxes. When set in motion in identical fashion the swing pairs kept in step, regardless of what material was in the boxes. Newton concluded that the mass and weight of the material are the same to an accuracy of at least 1 part in 100.
It was five years after Einstein had proposed his equivalence principle that he learned of some experiments, performed towards the end of the nineteenth century, which significantly improved upon Newton’s accuracy. The Hungarian physicist Roland von Eotvos had developed a device called a ‘torsion balance.' This consisted of a pair of objects of an equal mass attached to the opposite ends of a rod suspended by a fine wire. There is, of course, a gravitational force on each object, and, also, an inertia force due to the rotation of the Earth. If the gravitational and inertial masses are not exactly equal, the rod will rotate about a vertical axis until it is halted by the restoring torque of the twisted wire. The amount of twist is best measured by turning the whole apparatus, including the cable and its support, through 180 degrees. The rod will now spin in opposite direction. If two gravitational and inertia masses are equal, there will be no movement of the rod between the two orientations of the apparatus. Using this equipment, Eotvos was able to demonstrate their equality to within a few parts in 1000 million.
In the 1960s, groups, led by Robert Dicke from Princeton University in the United States of America, were able to improve on this result of Eotvos. Some ten years later, together with a Russian group headed by Vladimir Braginsky at Moscow State University, they were able to make a further improvement on the equality of two types of mass4. The accuracy of the balance now stands at about 1 part in 100,000 million. Less accurate, but still significant, experiments have also shown that molecules, neutrons, and electrons also fall exactly in step with dust particles and other objects. It is also interesting that technology has now improved sufficiently for the direct test of dropping two objects and comparing their descent to be as accurate as the more indirect Eotvos experiment one hundred years earlier. This new generation of tests force was motivated by the suggestion that gravity had an additional short-range force component that played no role in the large-scale astronomical manifestations of gravity. A variety of detailed experiments carried out between 1986 and 1990 now seem to rule out the existence of such an additional force.
Gravity is probably the most familiar of forces. We are acutely aware of it when climbing mountains, or even going up stairs. We are no longer surprised to see satellite pictures of astronauts floating in their space laboratories. Everything in the orbiting laboratory seems to be floating from drops of water to bottles of aftershave-since all objects are falling towards Earth with the same acceleration. It certainly looks as if gravity has been abolished. In fact, this is only approximately true. Imagine specks of dust sprinkled throughout the spacecraft. These specks nearest the Earth will be pulled towards the Earth slightly more sharply than those further away6. Since the probe is solid, it is falling towards the Earth at a standard rate. Inside the craft, the dust on the side nearest the Earth will fall towards ‘Earthside’ of the laboratory. Conversely, dust in the half of the ship furthest from the Earth will be seen to rise and settle on the ‘topside’ of the laboratory. In the case of ‘spaceship’ Earth in its orbit around the Sun, a similar effect takes place with the air and water, and this is the origin of the solar tides. The much larger lunar tides arise in the same way, from the effects resulting from the Moon orbiting the Earth. These residual effects of gravity in our freely falling laboratory are called ‘tidal forces’: as we make the laboratory smaller, the lower these tidal forces become and the closer we are to a real gravity-free situation. To describe the magnitude of such tidal forces entirely requires a full theory of gravity. To conclude the Einstein’s theory of gravity, it is necessary to explain some predictions that follow from the equivalence principle alone.
Having talked about the bending of light by gravity, and historically this was the first significant test of “Einstein’s new theory” 5. However, although the equivalence principle does not predict the bending of light, the full apparatus of general relativity is required to predict the magnitude of this deflection. In the absence of acceleration, the light will travel in a straight line; with the rocket accelerating upwards, the light is seen to bend. According to the equivalence principle, light must also be sent to a gravitational field. Einstein first attempted to calculate the magnitude of the bending of light by the Sun in 1911. In fact, although he did not know it at the time, he had obtained the same answer as one first achieved in 1803 by little-known Bavarian.
Works Cited
Ambrose, Lena. History of Gravity, Iowa. Rockwell City, Iowa: publisher not identified. 1970. Print.
Bennett, Jeffrey O. The Cosmic Perspective. Boston: Addison-Wesley, 2014. Print.
Clement, Gilles, and Angelia P. Bukley. Artificial Gravity. Hawthorne, Calif: Microcosm Press, 2007. Internet resource.
Clegg, Brian. Gravity: How the Weakest Force in the Universe Shaped Our Lives. New York: St. Martin's Press, 2012. Print.
Eisenstaedt, Jean, and Arturo Sangalli. The Curious History of Relativity: How Einstein's Theory of Gravity Was Lost and Found Again. Princeton, NJ [u.a.: Princeton Univ. Press, 2006. Print.
Hey, Anthony J. G, and Patrick Walters. Einstein's Mirror. New York: Cambridge University Press, 1996. Print.
Cite this page
Gravity Begins with Aristotle and Ends with Einstein, Free Essay. (2017, Sep 17). Retrieved from https://speedypaper.net/essays/gravity-begins-with-aristotle-and-ends-with-einstein
Request Removal
If you are the original author of this essay and no longer wish to have it published on the SpeedyPaper website, please click below to request its removal:
- Free Essay on Relating Temporal Cognition to Romanticism and Existentialism
- Maintaining your relationship
- The Alabama Music Hall of Fame - Business Description Essay Sample
- Free Essay with Phenom 100 and Cessna Citation Mustang Synopsis
- Characteristics of a Good Ruler, Essay Sample for Your Inspiration
- Essay Example: Mediation and Moderation
- Political Ideologies after WW1 and Great Depression - Essay Sample
Popular categories